A Novel Structure to Enhance Second Harmonic Generation in Plasmonic Waveguide
Keywords:
SHG, MGIM, plasmonics, Graphene, grating, asymmetric structureAbstract
The graphene plasmonic nano-cavity grating is a novel plasmonic structure that has been proven to significantly boost nonlinear optical second-harmonic production. In this article, we discuss how this structure works (SHG). In the suggested structure, metal niobate is positioned such that it is sandwiched between two distinct metals and a thin sheet of grating-patterned graphene. The combination of two distinct metals in a conductor has the potential to greatly amplify the nonlinear state of the conductor, which will lead to an increase in the specific heat capacity of the conductor. Graphene gratings connect the pump beam to two SPP waves that may cancel each other out, which results in the formation of a stationary SPP wave in the region between the gratings due to the mutual interference that occurs. The distance in between the two gratings will have its distance between them fine-tuned in order to improve the formation of second harmonics. It will be shown that field sweetening in proposed waveguides may result in significant improvements in SHG by optimizing the pure mathematics of the desired structure and using different metals. These two factors are meant to be done in conjunction with one another.
References
S. Abdollahramezani et al., "Tunable nanophotonics enabled by chalcogenide phase-change materials," Nanophotonics, vol. 9, pp. 1189-1241, 2020, doi: 10.1515/nanoph-2020-0039.
S. Abdollahramezani et al., "Dynamic hybrid metasurfaces," Nano Lett., vol. 21, pp. 1238-1245, 2021, doi: 10.1021/acs.nanolett.0c03625.
A. Ahmadivand and B. Gerislioglu, "Deep- and vacuum-ultraviolet metaphotonic light sources," Mater. Today, vol. 51, pp. 208-221, 2021, doi: 10.1016/j.mattod.2021.05.019.
J. Zhang, E. Cassan, D. Gao, and X. Zhang, "Highly efficient phase-matched second harmonic generation using an asymmetric plasmonic slot waveguide configuration in hybrid polymer-silicon photonics," Opt. Express, vol. 21, 2013, doi: 10.1364/OE.21.014876.
S. A. Maier, Plasmonics, Fundamentals and Applications. Springer, 2007.
R. X. Yang, R. A. Wahsheh, Z. L. Lu, and M. A. G. Abushagur, "Efficient light coupling between dielectric slot waveguide and plasmonic slot waveguide," Opt. Lett., vol. 35, pp. 649-651, 2010, doi: 10.1364/OL.35.000649.
P. Neutens, P. Van Dorpe, I. De Vlaminck, L. Lagae, and G. Borghs, "Electrical detection of confined gap plasmons in Metal-Graphene-insulator-Metal waveguides," Nat. Photonics, vol. 3, pp. 283-286, 2009, doi: 10.1038/nphoton.2009.47.
K. Wen et al., "Multiple Plasmon-Induced Transparency Responses in a Subwavelength Inclined Ring Resonators System," IEEE Photonics Journal, vol. 7, no. 6, pp. 1-7, 2015, doi: 10.1109/JPHOT.2015.2504966.
K. Wen, Y. Hu, L. Chen, J. Zhou, L. Lei, and Z. Guo, "Fano resonance with ultra-high figure of merits based on plasmonic Metal-Graphene-insulator-Metal waveguide," Plasmonics, vol. 10, no. 1, pp. 27-32, 2015, doi: 10.1007/s11468-014-9772-6.
K. Wen, Y. Hu, L. Chen, J. Zhou, L. Lei, and Z. Meng, "Single/dual Fano resonance based on plasmonic metal-dielectric-metal waveguide," Plasmonics, vol. 11, no. 1, pp. 315-321, 2016, doi: 10.1007/s11468-015-0056-6.
H. T. Miyazaki and Y. Kurokawa, "Squeezing visible light waves into a 3-nm-thick and 55-nm-long plasmon cavity," Physical Review Letters, vol. 96, p. 097401, 2006, doi: 10.1103/PhysRevLett.96.097401.
G. Veronis and S. Fan, "Bends and splitters in metal-dielectric-metal subwavelength plasmonic waveguides," Applied Physics Letters, vol. 87, p. 131102, 2005, doi: 10.1063/1.2056594.
Y. Kurokawa and H. T. Miyazaki, "Metal-Graphene-insulator-Metal plasmon nanocavities: Analysis of optical properties," Physical Review B, vol. 75, p. 035411, 2007, doi: 10.1103/PhysRevB.75.035411.
Y. Xie et al., "Theoretical investigation of a plasmonic sensor based on a metal-insulator-metal waveguide with a side-coupled nanodisk resonator," Journal of Nanophotonics, vol. 9, no. 1, 2015, doi: 10.1117/1.JNP.9.093099.
Y. Xie, Y. Huang, and W. Zhao, "A Novel Plasmonic Sensor Based on Metal-Insulator-Metal Waveguide With Side-Coupled Hexagonal Cavity," IEEE Photonics Journal, vol. 7, no. 2, 2015, doi: 10.1109/JPHOT.2015.2419635.
C. Zeng and Y. Cui, "Rainbow trapping of surface plasmon polariton waves in metal-insulator-metal graded grating waveguide," Optics Communications, vol. 290, pp. 188-191, 2013, doi: 10.1016/j.optcom.2012.10.020.
C. Zeng and Y. Cui, "Low-distortion plasmonic slow-light system at telecommunication regime," Optics Communications, vol. 294, pp. 372-376, 2013, doi: 10.1016/j.optcom.2012.12.037.
R. W. Boyd, Nonlinear Optics. Academic, 2008.
T. Harimoto, B. Yo, and K. Uchida, "A novel multipass scheme for enhancement of second harmonic generation," Opt. Express, vol. 19, 2011, doi: 10.1364/OE.19.022692.
C. G. Biris and N. C. Panoiu, "Second harmonic generation in metamaterials based on homogeneous centrosymmetric nanowires," Phys. Rev. B, vol. 81, no. 19, 2010, doi: 10.1103/PhysRevB.81.195102.
F. F. Lu, T. Li, X. P. Hu, Q. Q. Cheng, S. N. Zhu, and Y. Y. Zhu, "Efficient second harmonic generation in nonlinear plasmonic waveguide," Opt. Lett., vol. 36, 2011, doi: 10.1364/OL.36.003371.
S. Park, J. W. Hahn, and J. Y. Lee, "Doubly resonant metallic nanostructure for high conversion efficiency of second harmonic generation," Opt. Express, vol. 20, 2012, doi: 10.1364/OE.20.004856.
C. C. Neacsu, G. A. Reider, and M. B. Raschke, "Second harmonic generation from nanoscopic metal tips: symmetry selection rules for single asymmetric nanostructures," Phys. Rev. B, vol. 71, 2005, doi: 10.1103/PhysRevB.71.201402.
M. Cazzanelli et al., "Second-harmonic generation in silicon waveguides strained by silicon nitride," Nat. Mater., vol. 11, no. 2, pp. 148-154, 2011, doi: 10.1038/nmat3200.
J. S. Levy, M. A. Foster, A. L. Gaeta, and M. Lipson, "Harmonic generation in silicon nitride ring resonators," Opt. Express, vol. 19, no. 12, pp. 11415-11421, 2011, doi: 10.1364/OE.19.011415.
R. E. P. de Oliveira, M. Lipson, and C. J. S. de Matos, "Electrically controlled silicon nitride ring resonator for quasi-phase matched second-harmonic generation," in in CLEO: Science and Innovations, 2012: Optical Society of America, doi: 10.1364/CLEO_SI.2012.CF3M.5.
M. L. Brongersma and P. G. Kik, Surface Plasmon Nanophotonics. Springer, 2007.
A. R. Davoyan, I. V. Shadrivov, and Y. S. Kivshar, "Quadratic phase matching in nonlinear plasmonic nanoscale waveguides," Opt. Express, vol. 17, no. 22, pp. 20063-20068, 2009, doi: 10.1364/OE.17.020063.
W. S. Cai, A. P. Vasudev, and M. L. Brongersma, "Electrically controlled nonlinear generation of light with plasmonics," Science, vol. 333, no. 6050, pp. 1720-1723, 2011, doi: 10.1126/science.1207858.
S. B. Hasan, C. Rockstuhl, T. Pertsch, and F. Lederer, "Second-order nonlinear frequency conversion processes in plasmonic slot waveguides," J. Opt. Soc. Am. B, vol. 29, no. 7, pp. 1606-1611, 2012, doi: 10.1364/JOSAB.29.001606.
M. I. Stockman, "Nanoplasmonics: past, present, and glimpse into future," Opt. Express, vol. 19, no. 22, pp. 22029-22106, 2011, doi: 10.1364/OE.19.022029.
A. Yariv, "Phase conjugate optics and real-time holography," IEEE Journal of Quantum Electronics, vol. 14, no. 9, pp. 650-660, 1978, doi: 10.1109/JQE.1978.1069870.
H. J. Simon, D. E. Mitchell, and J. G. Watson, "Optical second harmonic generation with surface plasmons in silver films," Phys. Rev. Lett., vol. 33, 1974, doi: 10.1103/PhysRevLett.33.1531.
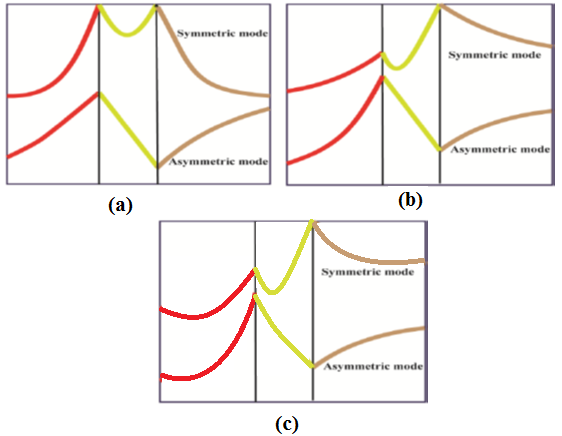